The Weekly Anthropocene Interviews: Andrew Adamatzky, Professor in Unconventional Computing
Andrew Adamatzky is Professor in Unconventional Computing at the University of the West of England, Bristol. He is a leading researcher in fungal computing, computing with other living substrates, and other novel hardware.
Professor Adamatzky’s research is at the cutting edge of integrating living organisms like fungi and slime mold with computers, in ways that have incredible potential for environmental data sensors and much else!
A lightly edited transcript of this exclusive interview follows. This writer’s questions and remarks are in bold, Professor Adamatzky’s responses are in regular type. Bold italics are clarifications and extra information added after the interview.
How did you get into fungal computing? What led you to this field of study?
My life time interest is to develop sensing and computing devices from unusual substrates. This is why I founded the Unconventional Computing Lab.
The Unconventional Computing Laboratory was founded by Prof Andy Adamatzky in 2001 as a response to an urgent need to develop computers for the next century. The computers of 2100+ will be made of living and chemical systems, they will be either purely ‘wet computers’ or hybrid computers where wetware, hardware and software co-work harmonically. [“Wet computers” and “wetware” here mean “involving living organisms.”]
Therefore, the lab employs complex dynamics in physical, chemical and biological media to design novel computational techniques, architectures and working prototypes of non-linear media based computers. [“Non-linear media based computers” here refers to computing with unusual “media,” like living fungi]. The research is based on an interdisciplinary cooperation in modelling and experimental verification of novel principles of information processing and analysis in uniform distributed large-scale chemical and physical systems. We apply these novel principles to the design of working prototypes of massively parallel computing devices with intrinsic functions that exploit the complex dynamics of nonlinear systems. [Nonlinear systems are systems in which “the change of the output is not proportional to the change of the input”-including many living organisms. For example, a mathematical equation like “y=2.5x” is linear: the output y will always be 2.5 times the input x. Nonlinear systems can undergo massive step-changes, like water transforming from liquid to gas at the boiling point of 100°C, or a living creature reacting differently to a stimulus after a certain threshold].
The Lab is proud of prototyping experimental laboratory computing devices. These include precipitating and oscillating chemical processors, oscillating chemical robotic controllers, crystallisation based processors, slime mould sensors and processors, swarms of soldier crabs logical circuits, intra-cellular computing, plant roots based processors, liquid computers, Belousov-Zhabotinsky medium based sensing and computing prototypes, experimental P-systems.
This writer had never heard of Belousov-Zhabotinsky reactions before this interview, but it’s really interesting: it’s a class of mixes of chemicals where complex reactions just keep happening for a really long time and “evolve chaotically,” occasionally self-organizing into complex patterns.
Recently, we got more results from analysis of electrical behaviour and properties of unconventional computing substrates, as evidenced by studies of oscillation behaviour of slime, resistance switches and oscillations of tectomers and tubulin microtubules, plant wires, artificial synapses with carbon nanotubes droplets, and, recently, sensing fungal skin.
Tectomers are a class of self-assembling molecules, while microtubules are tiny protein tubes that provide structure to eukaryotes’ cells-there are tons in your body as your read this!
How exactly can you interface between fungi as a computing substrate and traditional electronic computing equipment? Are there hyphae [little root-like tips of fungi that form mycelium] wrapped around tiny wires at some level? And how is that related to the electric "language" of fungi one of your recent papers discussed?
The electrical activity was measured with Pico ADC-24 [a data logger device]. The whole procedure was as follows.
I inserted electrodes in substrate colonised by fungal mycelium.
I observed action-potential like spiking. [Action potential, an event where the difference in electrical potential between the exterior and interior of a cell rises and falls rapidly, is a key way that brain, nerve, and other cells communicate. It’s exciting to see similar activity in fungi!].
I found that the spikes group in trains.
I collected and analysed statistics on train lengths.
I compared the distribution of the spikes' train lengths with the distribution of word lengths in human languages and found similarities.
Using probabilistic techniques I reconstructed potential syntax of fungal language.
This is more of a speculative question, but what do you think are the most interesting potential mid- and long-range technological possibilities in fungal computing? What amazing stuff could we make with this in the next few decades? Could we someday have fungus or slime-mould based "living adapters" allowing interfaces between mycorrhizal fungi networks [fungi living symbiotically with plants] and human-created electronic sensor systems? A live sensor update from the "Wood Wide Web" so to speak? A living "adapter" sensor that you could stick in the ground to connect your phone to nearby trees?
Fungal electronics represent a remarkable family of living electronic devices made from mycelium-bound composites or pure mycelium. These devices possess the unique ability to change their impedance and generate electrical spikes in response to external control parameters. Fungal electronics can be integrated into various applications, including fungal materials, wearables, and standalone sensing and computing devices.
Fungal Memristors: These devices, also known as Resistive Switching Devices (RSDs), exhibit a special property where their resistance is determined by internal variables. Memristors can achieve functionalities that traditional electronic components like resistors, capacitors, and inductors cannot, making them efficient for logical gate implementations.
[Memristors are a class of electronic components, an “electric resistor with memory.”]
Fungal Oscillators: Fungal oscillators convert direct current input into alternating current output by utilizing fluctuations in the electrical resistance of mycelium-bound composites. These oscillations are observed in nearly homogeneous mycelium sheets grown on suitable substrates.
Fungal Pressure Sensor: Fungal blocks can detect the application or removal of weight by generating distinct electrical spikes. The response to weight application differs from the response to weight removal, and these fungal responses exhibit habituation.
Fungal Photosensor: Living fungal materials respond to illumination by altering their electrical activity. This property enables their incorporation into logical circuits and actuators with optical inputs.
Fungal Analog Computing: Fungal analog computing is a numerical modeling and experimental approach that leverages the non-linear conductive properties of fungal substrates to implement logical mappings. This technique allows the representation of TRUE and FALSE values using voltage thresholds.
Practical applications of fungal electronics include sensorial and computing circuits embedded into mycelium-bound composites. For instance, the concept of reservoir computing can be employed to create mem-sensor devices from living fungi. While fungal electronic circuits may operate at a slower frequency than traditional electronics, they offer sustainability and self-powered operation. [This is a big plus-because fungi are living organisms, they can use the electricity from their bodies as a power source when integrated into electronics]. These living circuits can be utilized for tasks related to slowly changing parameters and hold potential in identifying the morphological and physiological state of various organic materials.
While mycelium network computing may not rival the established silicon architecture, its unique potential lies in specific application domains, particularly in the realm of living biosensors. Here, the distribution of gates can be influenced by environmental conditions, making it a valuable tool for real-time environmental monitoring and adaptation.
Additionally, mycelium network computing finds practical use in embedding computation within structural elements where fungal materials are employed. This opens doors to innovative implementations of fungal electronic properties. For instance, one approach involves harnessing reservoir computing for sensing, where the environment's data is encoded within the state of the reservoir memristive computing medium. This approach can be utilized to prototype sensing-memristive devices using living fungi as the foundation. Even though fungal electronic oscillators operate at very low frequencies, this characteristic doesn't preclude their integration into fully living or hybrid analog circuits that are embedded within fungal architectures.
Looking ahead, we envision the creation of specialized circuits and processors made from living fungi, enriched with functionalized nanoparticles. Prototypes of hybrid electronic devices using slime mold have already provided a glimpse into the possibilities. Potential applications for devices constructed from living fungi span a wide range, including environmental sensors seamlessly integrated into building structures and wearables. These devices can also serve as patches for monitoring various chemical parameters, offering a novel approach to human well-being and environmental awareness.
Can you tell me about Physarum polycephalum, your experiments with this species, its cognitive potential, and its ability to recreate highway networks?
P. polycephalum is an acellular slime mould. Its main vegetative phase is the plasmodium (the active streaming form of slime moulds). This is a single monstrously large cell with many diploid nuclei which behaves like an amoeba. In effect, the plasmodium behaves as a non-linear medium, an excitable soft matter, encapsulated in an elastic and growing membrane.
The cell is visible to the naked eye and can grow for many centimeters when properly cared for. When it is placed in an environment with distributed nutrients, it develops a network of protoplasmic tubes spanning the nutrients’ sources. The growing and feeding plasmodium exhibits characteristic rhythmic contractions with articulated sources. The contraction waves are associated with waves of potential change, and the waves observed in plasmodium are similar to the waves found in excitable chemical systems.
The slime mould is like a reaction-diffusion (and excitable) system encapsulated in an elastic growing membrane. Thus, we can use all the advantages of reaction-diffusion, wave-based computers, and also handle the computing substrate (slime mould) with ease.
When the plasmodium is placed on a substrate populated with sources of nutrients, it starts to explore the surrounding space. Numerous pseudopodia emerge, frequently branch, and proceed. These detect, by chemotaxis, the relative locations of the closest sources of nutrients. When another source of nutrients, element of the given planar set, is reached, the relevant part of the plasmodium reshapes and shrinks to a protoplasmic tube. This tube connects the initial and newly acquired sites and it represents an edge of the computed spanning tree. The plasmodium optimizes the network to efficiently transport protoplasm with nutrients.
To uncover analogies between biological and human-made transport networks and to project behavioural traits of biological networks onto development of vehicular transport networks. Motorway networks are designed for efficient vehicular transportation of goods and passengers, protoplasmic networks are developed for efficient intracellular transportation of nutrients and metabolites. Is there a similarity between these two networks?
We conducted a series of studies on slime mould’s evaluation and approximation of motorway networks, e.g. in Australia, Belgium, Germany, Italy, the UK, and USA (see, for example, a video of the slime mould exploring the USA.
We represented each region with an agar plate and imitated major urban areas with oat flakes. We inoculated plasmodium in a capital, then analysed the resulting structures of the developed protoplasmic networks. For all regions studied in laboratory experiments, we found that the network of protoplasmic tubes grown by plasmodium matches, at least partly, the network of human-made transport arteries.
We simplified the problem to a single transport route. In laboratory experiments with 3D Nylon terrains of the USA and Germany, we imitated the development of route 20 (the longest road in the USA) and autobahn 7 (the longest national motorway in Europe). We found that slime mould builds longer transport routes on 3D terrains, compared to flat agar plates, yet sufficiently approximates the man-made transport routes.
Usually it takes slime mould 4-5 days to cover the distance between Newport and Boston and 2-3 days to propagate from Flensburg to Fussen (such differences in propagation time are possibly due to the steep elevations in the West of USA, which prevent the slime mould from gaining speed and momentum when starting colonisation of the terrain). In experiments with Germany, one day of slime mould’s propagation roughly corresponds to 3-5 hours of real-life driving along the autobahn 7, and in experiments with USA to about 10 hours driving along route 20.
Which of your current/ongoing projects are you personally most excited about?
Computing with proteinoids. Proteinoids are thermal proteins which are produced by heating amino acids up to 140C. When placed in the water the proteinoids swell into microspheres. The microspheres produce spikes of electrical potential similar to that of real neurons. Thus the proteinoid microspheres are proto-neurons without metabolism. Thus we develop experimental approaches to do information processing and computation with ensembles of proteinoid microspheres.
What do you think will be the first fungal computing or slime mold computing technology to become commercially available? Will people see stuff like this on store shelves or be able to order home DIY kits in a decade, do you think, or will these technologies mostly be in research institutions for the foreseeable future?
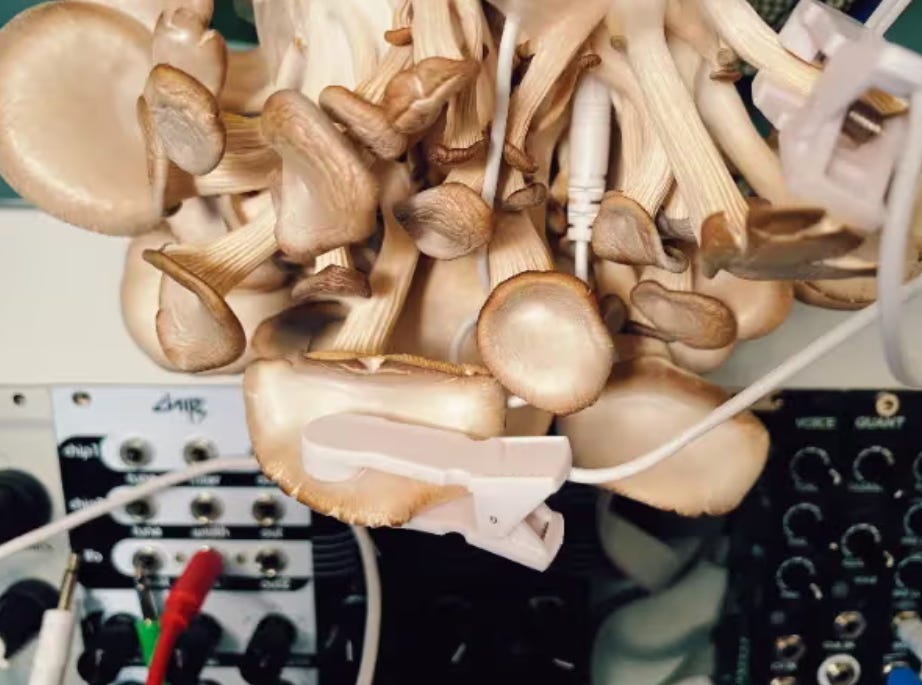
Thinking realistically, the first commercially available device would be a Mushroom Music Generator, this will comprise fungi growing kit, Arduino, speakers.
The second product will be fungal sensors, as above but without speakers but with a set of chemical to stimulate fungi with.
The third product will be a kit 'Generate any Boolean function with 8 inputs': an objective will be to -- somehow -- program fungi with chemical, electrical, optical, tactile stimuli to produce a predictable mapping of input binary strings (represented by impulses of electrical current) to output binary strings.
Wow, thank you for that fascinating and detailed prediction! It’s been a pleasure interviewing you.
Thanks. Warm regards.
Actually four ❤️❤️❤️❤️ likes warranted! This is research I am vitally interested in and I'm approaching computation using the rich repertoire of ant behavior. This interview is fascinating. Thanks Sam..another home run for you.